Because of lack of small pores it is difficult to separate small molecules with polymer monoliths in isocratic mode. We have prepared monolithic capillary columns and then hypercrosslinked them to afford a monolith containing an array of small pores [1].
This monolithic column affords good separation of uracil and alkylbenzenes in isocratic mobile phase mode (a column efficiency as high as 73 000 plates/m was determined for uracil) and also proved useful for separations in size exclusion mode.
Organic polymer monoliths and small molecules
Compare to silica based monoliths, porous polymer monoliths contain very small or even no concentration of small pores in their porous structure. Therefore, they exhibit much smaller surface areas (tens of square meter per gram) and usually are not suitable for separation of small molecules. Several approaches were explored to improve this drawback of organic polymer monoliths: copolymerization of dimethacrylates differing in the length and branching of the fragment connecting the polymerizable units[2]; the termination of the polymerization reaction at an early stage [3,4] to achieve large surface areas; and the use of high polymerization temperatures [5,6].
However, it has always proven difficult to prepare polymer monoliths possessing both large through pores and a multiplicity of small pores in a single step and alternative approaches needed to be developed.
Hypercrosslinking modification
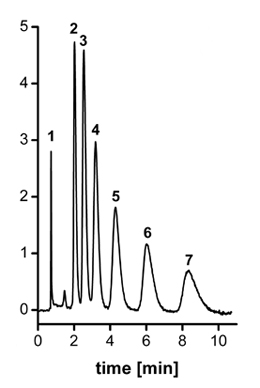
Hypercrosslinking, pioneered by Davankov several decades ago [7-10] enables the preparation of large surface area materials from preformed polymer precursors. The original implementation used linear polystyrene, which was cross-linked via Friedel-Crafts alkylation to afford materials containing mostly small pores [11].
The typical porous monolithic structure consisting of interconnected microglobules results from phase separation during polymerization of a mixture of monomers and porogens. For poly(styrene-co-vinylbenzyl chloride-co-divinylbenzene) monoliths less than ideal reactivity ratios for monomers such as styrene, chloromethylstyrene, and divinylbenzene lead to polymer microglobules amenable to hypercrosslinking. The divinyl monomer polymerizes faster, and the remaining monomer mixture becomes significantly richer in the monovinyl monomers as the polymerization reaction nears completion. This mixture then affords only slightly cross-linked chains attached to the surface of highly crosslinked microglobular scaffolds. When the pores are filled with a thermodynamically good solvent such as 1,2-dichloroethane, this surface polymer layer is solvated.
Capillary liquid chromatography
The precursor column performs poorly as all alkylbenzenes are less retained and eluted in a single broad peak. In contrast, baseline separation of all alkylbenzenes is obtained with the column after hypercrosslinking (see Figure). On the other hand, gradient separation of the proteins is better on the non-modified column because of negative effect of the small pores on the gradient separation [12]. Finally, because of significant concentration of small pores, these columns can be used for separation of polymers in size-exclusion chromatography.
Our work clearly demonstrates the possibility of postpolymerization hypercrosslinking of the monolithic stationary phase to afford columns for efficient isocratic separation of small molecules in reversed phase and polymers in size exclusion modes.
References
- Urban, J., Svec, F., Fréchet, J.M.J. Anal. Chem. 2010, 82.
- Xu, Z., Yang, L. and Wang, Q. J. Chromatogr. A 2009, 1216, 3098 – 3106.
- Wang, Q., Svec, F. and Fréchet, J. M. J. Anal. Chem. 1995, 67, 670 – 674.
- Trojer, L., Bisjak, C. P., Wieder, W. and Bonn, G. K. J. Chromatogr. A 2009, 1216, 6303 – 6307.
- Peters, E. C., Svec, F. and Fréchet, J. M. J. Adv. Mater. 1999, 11, 1169 – 1181
- Meyer, U., Svec, F., Fréchet, J. M. J., Hawker, C. J. and Irgum, K. Macromolecules 2000, 33, 7769 – 7775.
- Davankov, V. A., Rogozhin, S. V. and Tsyurupa, M. P. Macronet Polystyrene Structures for Ionites and Method of Producing Same. U.S. Patent 3,729,457, April 24, 1973.
- Pastukhov, A. V., Tsyurupa, M. P. and Davankov, V. A. J. Polym. Sci., Polym. Phys. 1999, 37, 2324 – 33.
- Davankov, V. A. and Tsyurupa, M. P. React. Polym. 1990, 13, 27 – 42.
- Davankov, V. A., Tsyurupa, M., Ilyin, M. and Pavlova, L. J. Chromatogr. A 2002, 965, 65 – 73.
- Tsyurupa, M. P. and Davankov, V. A. React. Funct. Polym. 2006, 66, 768 – 779.
- Urban, J., Moravcova, D. and Jandera, P. J. Sep. Sci. 2006, 29, 1064– 73